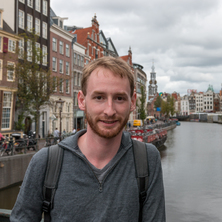
Overview
Background
Working in theoretical atomic physics and particle astrophysics. My research focusses on high-precision atomic structure calculations, and how atomic processes can be used for testing fundamental theories, probing for physics beyond the standard model, and searching for dark matter. This is complimentary to the high-energy tests performed at CERN. Some research highlights include: searching for variations in the fundamental constants near the super-massive black hole at the centre of our galaxy [1]; using decades of archived atomic clock data from the GPS satellites to search for signatures of dark matter [2]; performing high-precision calculations of symmetry violations in atoms, allowing the most precise low-energy test of the standard model to date [3-5]; and proposing and quantifying novel experimental signatures of dark matter that exploit atomic (rather than the typical nuclear) phenomena, opening the door to a wide range of previously “invisible” models [6-9].
- A. Hees, T. Do, B. M. Roberts, A. M. Ghez, S. Nishiyama, R. O. Bentley, A. K. Gautam, S. Jia, T. Kara, J. R. Lu, H. Saida, S. Sakai, M. Takahashi, and Y. Takamori, Search for a Variation of the Fine Structure Constant around the Supermassive Black Hole in Our Galactic Center, Phys. Rev. Lett. 124, 081101 (2020).
- B. M. Roberts, G. Blewitt, C. Dailey, M. Murphy, M. Pospelov, A. Rollings, J. Sherman, W. Williams, and A. Derevianko, Search for Domain Wall Dark Matter with Atomic Clocks on Board Global Positioning System Satellites, Nature Comm. 8, 1195 (2017).
- V. A. Dzuba, J. C. Berengut, V. V. Flambaum, and B. M. Roberts, Revisiting Parity Nonconservation in Cesium, Phys. Rev. Lett. 109, 203003 (2012).
- B. M. Roberts and J. S. M. Ginges, Nuclear Magnetic Moments of Francium-207–213 from Precision Hyperfine Comparisons, Phys. Rev. Lett. 125, 063002 (2020).
- G. Sanamyan, B. M. Roberts, and J. S. M. Ginges, Empirical Determination of the Bohr-Weisskopf Effect in Cesium and Improved Tests of Precision Atomic Theory in Searches for New Physics, Phys. Rev. Lett. 130, 053001 (2023).
- B. M. Roberts, Y. V. Stadnik, V. A. Dzuba, V. V. Flambaum, N. Leefer, and D. Budker, Limiting P-Odd Interactions of Cosmic Fields with Electrons, Protons, and Neutrons, Phys. Rev. Lett. 113, 081601 (2014).
- B. M. Roberts, V. V. Flambaum, and G. F. Gribakin, Ionization of Atoms by Slow Heavy Particles, Including Dark Matter, Phys. Rev. Lett. 116, 023201 (2016).
- B. M. Roberts et al., Search for Transient Variations of the Fine Structure Constant and Dark Matter Using Fiber-Linked Optical Atomic Clocks, New J. Phys. 22, 093010 (2020).
- E. Savalle, A. Hees, F. Frank, E. Cantin, P.-E. Pottie, B. M. Roberts, L. Cros, B. T. McAllister, and P. Wolf, Searching for Dark Matter with an Optical Cavity and an Unequal-Delay Interferometer, Phys. Rev. Lett. 126, 051301 (2021).
Availability
- Dr Benjamin Roberts is:
- Available for supervision
- Media expert
Fields of research
Qualifications
- Doctor of Philosophy, University of New South Wales
Research interests
-
Atomic structure theory
-
Low-energy tests of fundamental physics
-
Dark matter
-
Particle astrophysics
Works
Search Professor Benjamin Roberts’s works on UQ eSpace
Featured
2023
Journal Article
Empirical determination of the Bohr-Weisskopf effect in cesium and improved tests of precision atomic theory in searches for new physics
Sanamyan, G., Roberts, B. M. and Ginges, J. S. M. (2023). Empirical determination of the Bohr-Weisskopf effect in cesium and improved tests of precision atomic theory in searches for new physics. Physical Review Letters, 130 (5) 053001, 1-6. doi: 10.1103/physrevlett.130.053001
Featured
2021
Journal Article
Searching for dark matter with an optical cavity and an uequal-delay interferometer
Savalle, Etienne, Hees, Aurélien, Frank, Florian, Cantin, Etienne, Pottie, Paul-Eric, Roberts, Benjamin M., Cros, Lucie, McAllister, Ben T. and Wolf, Peter (2021). Searching for dark matter with an optical cavity and an uequal-delay interferometer. Physical Review Letters, 126 (5) 051301, 051301. doi: 10.1103/physrevlett.126.051301
Featured
2020
Journal Article
Nuclear magnetic moments of Francium-207–213 from precision hyperfine comparisons
Roberts, B. M. and Ginges, J. S. M. (2020). Nuclear magnetic moments of Francium-207–213 from precision hyperfine comparisons. Physical Review Letters, 125 (6) 063002, 063002. doi: 10.1103/physrevlett.125.063002
Featured
2020
Journal Article
Search for a variation of the fine structure constant around the supermassive black hole in our Galactic Center
Hees, A., Do, T., Roberts, B. M., Ghez, A. M., Nishiyama, S., Bentley, R. O., Gautam, A. K., Jia, S., Kara, T., Lu, J. R., Saida, H., Sakai, S., Takahashi, M. and Takamori, Y. (2020). Search for a variation of the fine structure constant around the supermassive black hole in our Galactic Center. Physical Review Letters, 124 (8) 081101, 081101. doi: 10.1103/physrevlett.124.081101
Featured
2019
Journal Article
Electron-interacting dark matter: implications from DAMA/LIBRA-phase2 and prospects for liquid xenon detectors and NaI detectors
Roberts, B. M. and Flambaum, V. V. (2019). Electron-interacting dark matter: implications from DAMA/LIBRA-phase2 and prospects for liquid xenon detectors and NaI detectors. Physical Review D, 100 (6) 063017. doi: 10.1103/physrevd.100.063017
Featured
2017
Journal Article
Search for domain wall dark matter with atomic clocks on board global positioning system satellites
Roberts, Benjamin M., Blewitt, Geoffrey, Dailey, Conner, Murphy, Mac, Pospelov, Maxim, Rollings, Alex, Sherman, Jeff, Williams, Wyatt and Derevianko, Andrei (2017). Search for domain wall dark matter with atomic clocks on board global positioning system satellites. Nature Communications, 8 (1) 1195. doi: 10.1038/s41467-017-01440-4
Featured
2016
Journal Article
Ionization of Atoms by Slow Heavy Particles, Including Dark Matter
Roberts, B. M., Flambaum, V. V. and Gribakin, G. F. (2016). Ionization of Atoms by Slow Heavy Particles, Including Dark Matter. Physical Review Letters, 116 (2) e023201. doi: 10.1103/physrevlett.116.023201
Featured
2014
Journal Article
Limiting P-odd interactions of cosmic fields with electrons, protons, and neutrons
Roberts, B. M., Stadnik, Y. V., Dzuba, V. A., Flambaum, V. V., Leefer, N. and Budker, D (2014). Limiting P-odd interactions of cosmic fields with electrons, protons, and neutrons. Physical Review Letters, 113 (8) 081601, 081601. doi: 10.1103/physrevlett.113.081601
Featured
2012
Journal Article
Revisiting Parity Nonconservation in Cesium
Dzuba, V. A., Berengut, J. C., Flambaum, V. V. and Roberts, B. (2012). Revisiting Parity Nonconservation in Cesium. Physical Review Letters, 109 (20) 203003. doi: 10.1103/physrevlett.109.203003
2023
Journal Article
Accurate electron-recoil ionization factors for dark matter direct detection in xenon, krypton, and argon
Caddell, A. R., Flambaum, V. V. and Roberts, B. M. (2023). Accurate electron-recoil ionization factors for dark matter direct detection in xenon, krypton, and argon. Physical Review D, 108 (8) 083030, 1-10. doi: 10.1103/physrevd.108.083030
2023
Journal Article
Electric-dipole transition amplitudes for atoms and ions with one valence electron
Roberts, B. M., Fairhall, C. J. and Ginges, J. S. M. (2023). Electric-dipole transition amplitudes for atoms and ions with one valence electron. Physical Review A, 107 (5) 052812. doi: 10.1103/physreva.107.052812
2023
Journal Article
Experimental and theoretical study of dynamic polarizabilities in the 5S1/2 – 5D5/2 clock transition in rubidium-87 and determination of electric dipole matrix elements
Hamilton, Rhona, Roberts, Benjamin M., Scholten, Sarah K., Locke, Clayton, Luiten, Andre N., Ginges, Jacinda S.M. and Perrella, Christopher (2023). Experimental and theoretical study of dynamic polarizabilities in the 5S1/2 – 5D5/2 clock transition in rubidium-87 and determination of electric dipole matrix elements. Physical Review Applied, 19 (5) 054059. doi: 10.1103/physrevapplied.19.054059
2023
Journal Article
QED radiative corrections to electric dipole amplitudes in heavy atoms
Fairhall, C. J., Roberts, B. M. and Ginges, J. S. M. (2023). QED radiative corrections to electric dipole amplitudes in heavy atoms. Physical Review A, 107 (2) 022813. doi: 10.1103/physreva.107.022813
2022
Journal Article
Bohr-Weisskopf effect: from hydrogenlike-ion experiments to heavy-atom calculations of the hyperfine structure
Roberts, B. M., Ranclaud, P. G. and Ginges, J. S. M. (2022). Bohr-Weisskopf effect: from hydrogenlike-ion experiments to heavy-atom calculations of the hyperfine structure. Physical Review A, 105 (5) 052802. doi: 10.1103/physreva.105.052802
2022
Journal Article
Comment on “New physics constraints from atomic parity violation in 133Cs ”
Roberts, B. M. and Ginges, J. S. M. (2022). Comment on “New physics constraints from atomic parity violation in 133Cs ”. Physical Review D, 105 (1) 018301. doi: 10.1103/physrevd.105.018301
2021
Journal Article
Hyperfine anomaly in heavy atoms and its role in precision atomic searches for new physics
Roberts, B. M. and Ginges, J. S. M. (2021). Hyperfine anomaly in heavy atoms and its role in precision atomic searches for new physics. Physical Review A, 104 (2) 022823. doi: 10.1103/physreva.104.022823
2021
Journal Article
Precision measurement noise asymmetry and its annual modulation as a dark matter signature
Roberts, Benjamin M. and Derevianko, Andrei (2021). Precision measurement noise asymmetry and its annual modulation as a dark matter signature. Universe, 7 (3) 50, 50. doi: 10.3390/universe7030050
2021
Conference Publication
The DAMNED experiment ! New constraints on ultralight dark matter scalar field oscillations
Savalle, Etienne, Hees, Aurélien, Frank, Florian, Cantin, Etienne, Pottie, Paul-Eric, Roberts, Benjamin M., Cros, Lucie, McAllister, Ben T. and Wolf, Peter (2021). The DAMNED experiment ! New constraints on ultralight dark matter scalar field oscillations. 55th Rencontres de Moriond - 2021 Gravitation, Virtual, 9-11 March 2021. ARISF.
2020
Journal Article
Search for transient variations of the fine structure constant and dark matter using fiber-linked optical atomic clocks
Roberts, Benjamin M, Delva, Pacome, Al-Masoudi, Ali, Amy-Klein, Anne, Baerentsen, Christian, Baynham, Charles, Benkler, Erik, Bilicki, Sŀawomir, Bize, Sebastien, Bowden, William, Calvert, J, Cambier, Valentin, Cantin, Etienne, Curtis, Elizabeth Anne, Dörscher, Sören, Favier, Maxime, Frank, Florian, Gill, Patrick, Godun, R M, Grosche, Gesine, Guo, Changlei, Hees, Aurelien, Hill, Ian Robert, Hobson, Richard, Huntemann, Nils, Kronjaeger, Jochen, Koke, Sebastian, Kuhl, Alexander, Lange, Richard ... Wolf, Peter (2020). Search for transient variations of the fine structure constant and dark matter using fiber-linked optical atomic clocks. New Journal of Physics, 22 (9) 093010, 093010. doi: 10.1088/1367-2630/abaace
2020
Journal Article
Erratum: Calculations of the atomic structure for the low-lying states of actinium [Phys. Rev. A 100 , 022504 (2019)]
Dzuba, V. A., Flambaum, V. V. and Roberts, B. M. (2020). Erratum: Calculations of the atomic structure for the low-lying states of actinium [Phys. Rev. A 100 , 022504 (2019)]. Physical Review A, 101 (5) 059901. doi: 10.1103/physreva.101.059901
Funding
Current funding
Supervision
Availability
- Dr Benjamin Roberts is:
- Available for supervision
Before you email them, read our advice on how to contact a supervisor.
Available projects
-
Development of high-accuracy atomic physics methods
High-precision atomic physics experiments play an important role in testing the Standard Model of particle physics at low energy. Highly accurate atomic structure calculations are required in order to interpret the experiments in terms of fundamental physics parameters. Atomic physics calculations involve treating the many-electron atomic Hamiltonian approximately. In order to achieve high accuracy, a number of many-body effects need to be taken into account using perturbation theory. This project is to continue to develop and test techniques for extending many-body methods for high-precision calculations of atomic systems.
Atomic physics calculations involve treating the many-electron atomic Hamiltonian approximately. In order to achieve high accuracy, a number of many-body effects need to be taken into account using perturbation theory. In particular, one such class of effects, known as "ladder diagrams", are missing from some calculations. Though small, these corrections seem to be important in some cases. The ladder-diagram method has been applied previously to energies with high success (see: Physical Review A, 78, 042502.) The plan here is to extend this method to include "ladder diagram" corrections directly into atomic wavefunctions. These wavefunctions can then be used to compute relevant atomic properties (for example, hyperfine splittings, transition rates, lifetimes etc.). The project will involve aspects of quantum mechanics (elementary atomic theory) and numerical methods (application of existing code libraries to new problems in atomic physics). It will also involve some basic programming (in c++ and/or fortran), though no prior knowledge of programming is required.
-
-
Theoretical characterisation of systems for the development of atomic clocks
Atomic clocks are the most precise instruments ever constructed. They work by locking the frequency of radiation source (e.g., a laser) to the resonance of an atomic transition.
As well as the canonical application of clocks (positioning, navigation, and timing), their unparalleled stability makes them exceptional tools for fundamental physics studies as well. Atomic clocks may be used to: - test quantum mechanics with extraordinary precision - probe for evidence of exotic physics, such as violations of the equivalence principle - search for or constrain possible variations of the fundamental constants - search for dark matter and dark energy
It is currently an exciting time for atomic clocks, with the best optical atomic clocks approaching a staggering precision of parts in 10^20! There are many proposals to develop new optical atomic clocks in alkali atoms (Rb, Cs) and alkali-like ions (Sr+, Ba+, Ra+). In order to develop the most accurate atomic clocks, a thorough theoretical understanding of the atomic properties is required. This may be achieved by calculating the atomic wavefunctions using highly-precise atomic structure methods, which will be used and developed in this project. Quantities that must be calculated include atomic spectra, lifetimes of excited states, atomic polarisabilities, transition rates, light shifts (the perturbation of atomic levels by the lasers required for the clock operation), black-body radiation shifts, and many others.
Sensitivity coefficients, which determine how sensitive a particular clock transition is to variations in the fine-structure constants, will also be calculated.
-
-
Enlightening the search for dark matter (and other exotic physics)
Despite overwhelming astronomical and cosmological evidence for its existence, the microscopic composition of dark matter remains a complete mystery. The vast majority of the effort to detect dark matter has focused on weakly interacting massive particles (WIMPs), with masses ~10 – 1000 GeV. WIMPs in that mass range may scatter off atomic nuclei leaving detectable recoil energy in experiments. However, this represents only a tiny sliver of the possible mass range for dark matter particles, which may have masses down to < ~ 10^(-20) eV. Current experiments are blind to the majority of this parameter space. Taking advantage of atomic (rather than nuclear) phenomena, however, may drastically increase the range of masses we can search for.
As the dark matter (DM) mass drops much below the nucleus mass (<GeV), no appreciable nuclear recoils are observable. However, DM particles may instead scatter of atomic electrons, leading to observable ionisations. As the DM mass drops below that of the electron (<~MeV), there are no detectable electron recoils, however, a detectable signal may instead come from DM absorption ("dark" photoelectric effect). Finally, as the DM mass drops below the ~eV scale, it behaves as a classical radiation field. Then, the observable effect comes in the form of atomic interactions with the classical DM field, such as energy shifts caused by dark-matter-induced effective variation of fundamental constants.
Combining ideas from particle astrophysics with theoretical atomic physics, this project is to identify and quantify new observable effects that can be used to search for evidence of new particles and fields, which may be due to dark matter, dark energy, or something even more exotic. In particular, it will involve investigating and calculating rates of scattering, absorption, energy-level shifts in atomic systems.
-
Relativistic effects in atomic structure theory
In heavy atomic systems, the electron wavefunction must be treated relativistically (Dirac equation) to accurately calculate atomic properties. The inter-electron Coulomb interaction, however, is still typically treated using a non-relativistic formalism. The lowest-order relativistic correction to the electron-electron repulsion is given by the Breit Hamiltonian (see, e.g., Ref. [1]), which accounts for magnetic and finite-speed-of-light effects.
This typically leads to only very small correction. However, for certain transitions in ionised atoms, the relativistic effects are expected to be greatly enhanced. This project would involve calculating Breit corrections to highly-charged ions, to determine if the lowest-order Breit correction is sufficient, or if new methods must be developed.
A strong theoretical understanding of the structure of ions is important for a number of applications, including high-precision tests of the Standard Model and electroweak theory at low energy, and searches for dark matter and exotic physics. Highly-charged ions are also great candidates for next-generating atomic clocks, and highly-accurate theoretical calculations are required to aid in their development.
[1] Bethe and Salpeter, Quantum Mechanics of One-and Two-Electron Atoms (1977).
-
-
Atomic physics as a probe of the Standard Model
The Standard Model is extremely effective at describing the fundamental particles and interactions, but is widely believed to be incomplete. For example, the Standard Model cannot explain the asymmetry between matter and antimatter, and there is so far no leading candidate for a theory of dark matter or dark energy. Recent advances in both theory and experiment for atomic physics have opened a number of new and exciting avenues for discovery, and have placed this field at the forefront of probing fundamental physics and searching for dark matter. Further, the different energy scales involved in atomic processes make such experiments sensitive to a different range of new physics signatures than conventional searches, such as those at largescale high-energy physics experiments, like colliders at CERN, or large underground neutrino detectors. Input is needed from atomic theory both to interpret the results of experiments in terms of new physics theories, and to direct future experiments.
In particular, high-precision atomic physics experiments play an important role in testing the Standard Model of particle physics at low energy. Highly accurate atomic structure calculations are required in order to interpret the experiments in terms of fundamental physics parameters. Atomic physics calculations involve treating the many-electron atomic Hamiltonian approximately. In order to achieve high accuracy, a number of many-body effects need to be taken into account using perturbation theory. This project is to continue to develop and test techniques for extending many-body methods for high-precision calculations of atomic systems with the aim of extending and improving atomic probes of fundamental physics.
-
-
Dark matter induced atomic ionisation
Develop and test atomic methods for calculating dark matter interactions with atoms. This includes atomic excitation and ionisation caused by the scattering of dark matter particles by atoms, as well as the absoroption of dark matter particles by atoms (so-called dark photo-electric effect).
The project will involve aspects of quantum mechanics (elementary atomic theory, scattering theory) and particle astrophysics (application to dark matter direct detection experiments, and interpretation of results in terms of dark matter and particle physics models). It will also involve some basic programming (in c++ and/or python), though no prior knowledge of programming is required.
For some details, see: * "Electron-interacting dark matter: Implications from DAMA/LIBRA-phase2 and prospects for liquid xenon detectors and NaI detectors." Physical Review D, 100, 063017 (2019) [http://arxiv.org/abs/1904.07127]
-
-
Atomic physics as a probe of the Standard Model
The Standard Model is extremely effective at describing the fundamental particles and interactions, but is widely believed to be incomplete. For example, the Standard Model cannot explain the asymmetry between matter and antimatter, and there is so far no leading candidate for a theory of dark matter or dark energy. Recent advances in both theory and experiment for atomic physics have opened a number of new and exciting avenues for discovery, and have placed this field at the forefront of probing fundamental physics and searching for dark matter. Further, the different energy scales involved in atomic processes make such experiments sensitive to a different range of new physics signatures than conventional searches, such as those at largescale high-energy physics experiments, like colliders at CERN, or large underground neutrino detectors. Input is needed from atomic theory both to interpret the results of experiments in terms of new physics theories, and to direct future experiments.
In particular, high-precision atomic physics experiments play an important role in testing the Standard Model of particle physics at low energy. Highly accurate atomic structure calculations are required in order to interpret the experiments in terms of fundamental physics parameters. Atomic physics calculations involve treating the many-electron atomic Hamiltonian approximately. In order to achieve high accuracy, a number of many-body effects need to be taken into account using perturbation theory. This project is to continue to develop and test techniques for extending many-body methods for high-precision calculations of atomic systems with the aim of extending and improving atomic probes of fundamental physics.
-
-
Dark matter induced atomic ionisation
Develop and test atomic methods for calculating dark matter interactions with atoms. This includes atomic excitation and ionisation caused by the scattering of dark matter particles by atoms, as well as the absoroption of dark matter particles by atoms (so-called dark photo-electric effect).
The project will involve aspects of quantum mechanics (elementary atomic theory, scattering theory) and particle astrophysics (application to dark matter direct detection experiments, and interpretation of results in terms of dark matter and particle physics models). It will also involve some basic programming (in c++ and/or python), though no prior knowledge of programming is required.
For some details, see: * "Electron-interacting dark matter: Implications from DAMA/LIBRA-phase2 and prospects for liquid xenon detectors and NaI detectors." Physical Review D, 100, 063017 (2019) [http://arxiv.org/abs/1904.07127]
-
-
Theoretical characterisation of systems for the development of atomic clocks
Atomic clocks are the most precise instruments ever constructed. They work by locking the frequency of radiation source (e.g., a laser) to the resonance of an atomic transition.
As well as the canonical application of clocks (positioning, navigation, and timing), their unparalleled stability makes them exceptional tools for fundamental physics studies as well. Atomic clocks may be used to: - test quantum mechanics with extraordinary precision - probe for evidence of exotic physics, such as violations of the equivalence principle - search for or constrain possible variations of the fundamental constants - search for dark matter and dark energy
It is currently an exciting time for atomic clocks, with the best optical atomic clocks approaching a staggering precision of parts in 10^20! There are many proposals to develop new optical atomic clocks in alkali atoms (Rb, Cs) and alkali-like ions (Sr+, Ba+, Ra+). In order to develop the most accurate atomic clocks, a thorough theoretical understanding of the atomic properties is required. This may be achieved by calculating the atomic wavefunctions using highly-precise atomic structure methods, which will be used and developed in this project. Quantities that must be calculated include atomic spectra, lifetimes of excited states, atomic polarisabilities, transition rates, light shifts (the perturbation of atomic levels by the lasers required for the clock operation), black-body radiation shifts, and many others.
Sensitivity coefficients, which determine how sensitive a particular clock transition is to variations in the fine-structure constants, will also be calculated.
-
-
Development of high-accuracy atomic physics methods
High-precision atomic physics experiments play an important role in testing the Standard Model of particle physics at low energy. Highly accurate atomic structure calculations are required in order to interpret the experiments in terms of fundamental physics parameters. Atomic physics calculations involve treating the many-electron atomic Hamiltonian approximately. In order to achieve high accuracy, a number of many-body effects need to be taken into account using perturbation theory. This project is to continue to develop and test techniques for extending many-body methods for high-precision calculations of atomic systems.
Atomic physics calculations involve treating the many-electron atomic Hamiltonian approximately. In order to achieve high accuracy, a number of many-body effects need to be taken into account using perturbation theory. In particular, one such class of effects, known as "ladder diagrams", are missing from some calculations. Though small, these corrections seem to be important in some cases. The ladder-diagram method has been applied previously to energies with high success (see: Physical Review A, 78, 042502.) The plan here is to extend this method to include "ladder diagram" corrections directly into atomic wavefunctions. These wavefunctions can then be used to compute relevant atomic properties (for example, hyperfine splittings, transition rates, lifetimes etc.). The project will involve aspects of quantum mechanics (elementary atomic theory) and numerical methods (application of existing code libraries to new problems in atomic physics). It will also involve some basic programming (in c++ and/or fortran), though no prior knowledge of programming is required.
-
-
Enlightening the search for dark matter (and other exotic physics)
Despite overwhelming astronomical and cosmological evidence for its existence, the microscopic composition of dark matter remains a complete mystery. The vast majority of the effort to detect dark matter has focused on weakly interacting massive particles (WIMPs), with masses ~10 – 1000 GeV. WIMPs in that mass range may scatter off atomic nuclei leaving detectable recoil energy in experiments. However, this represents only a tiny sliver of the possible mass range for dark matter particles, which may have masses down to < ~ 10^(-20) eV. Current experiments are blind to the majority of this parameter space. Taking advantage of atomic (rather than nuclear) phenomena, however, may drastically increase the range of masses we can search for.
As the dark matter (DM) mass drops much below the nucleus mass (<GeV), no appreciable nuclear recoils are observable. However, DM particles may instead scatter of atomic electrons, leading to observable ionisations. As the DM mass drops below that of the electron (<~MeV), there are no detectable electron recoils, however, a detectable signal may instead come from DM absorption ("dark" photoelectric effect). Finally, as the DM mass drops below the ~eV scale, it behaves as a classical radiation field. Then, the observable effect comes in the form of atomic interactions with the classical DM field, such as energy shifts caused by dark-matter-induced effective variation of fundamental constants.
Combining ideas from particle astrophysics with theoretical atomic physics, this project is to identify and quantify new observable effects that can be used to search for evidence of new particles and fields, which may be due to dark matter, dark energy, or something even more exotic. In particular, it will involve investigating and calculating rates of scattering, absorption, energy-level shifts in atomic systems.
-
Relativistic effects in atomic structure theory
In heavy atomic systems, the electron wavefunction must be treated relativistically (Dirac equation) to accurately calculate atomic properties. The inter-electron Coulomb interaction, however, is still typically treated using a non-relativistic formalism. The lowest-order relativistic correction to the electron-electron repulsion is given by the Breit Hamiltonian (see, e.g., Ref. [1]), which accounts for magnetic and finite-speed-of-light effects.
This typically leads to only very small correction. However, for certain transitions in ionised atoms, the relativistic effects are expected to be greatly enhanced. This project would involve calculating Breit corrections to highly-charged ions, to determine if the lowest-order Breit correction is sufficient, or if new methods must be developed.
A strong theoretical understanding of the structure of ions is important for a number of applications, including high-precision tests of the Standard Model and electroweak theory at low energy, and searches for dark matter and exotic physics. Highly-charged ions are also great candidates for next-generating atomic clocks, and highly-accurate theoretical calculations are required to aid in their development.
[1] Bethe and Salpeter, Quantum Mechanics of One-and Two-Electron Atoms (1977).
-
Supervision history
Current supervision
-
Doctor Philosophy
Exploring possibilities for dark matter detection via atomic interactions
Principal Advisor
Other advisors: Associate Professor Jacinda Ginges
-
Doctor Philosophy
Tests of fundamental physics in atoms
Associate Advisor
Other advisors: Associate Professor Jacinda Ginges
-
Doctor Philosophy
High-precision atomic theory and searches for new physics
Associate Advisor
Other advisors: Associate Professor Jacinda Ginges
-
Doctor Philosophy
Searching for new physics in precision atomic experiments
Associate Advisor
Other advisors: Associate Professor Jacinda Ginges
Media
Enquiries
Contact Dr Benjamin Roberts directly for media enquiries about:
- Atomic clock
- Atomic physics
- Dark matter
- particle astrophysics
Need help?
For help with finding experts, story ideas and media enquiries, contact our Media team: